Fact Sheet
Funding Body | European Research Council (ERC) |
Funding Programme | Horizon Europe |
Funding Call | ERC Advanced Grants 2021 |
Acronym | qMOTION |
Full Title | Simulation-enhanced Highdensity Magneto-myographic Quantum Sensor Systems for Decoding Neuromuscular Control During Motion |
Awarded Funding | 3.5 Million Euros for 5 years |
Funding ID | ERC-AdG 2021 #101055186 |
Current Status | Preperation of Grant Agreement |
Starting Date | (Tentative) September 1, 2022 |
Abstract
Being able to decode neural signals that control skeletal muscles with high accuracy will enable scientific breakthroughs in diagnostics and treatment, including early detection of neurodegenerative diseases, optimising personalised treatment or gene therapy, and assistive technologies like neuroprostheses. This breakthrough will require technology that is able to record signals from skeletal muscles in sufficient detail to allow the morpho-functional state of the neuromuscular system to be extracted. No existing technology can do this. Measuring the magnetic field induced by the flow of electrical charges in skeletal muscles, known as Magneto-myography (MMG), is expected to be the game-changing technology because magnetic fields are not attenuated by biological tissue. However, the extremely small magnetic fields involved require extremely sensitive magnetometers. The only promising option is novel quantum sensors, such as optically pumped magnetometers (OPMs), because they are small, modular, and can operate outside of specialised rooms. Our vision is to use this technology and our expertise in computational neuromechanics to decode, for the first time, neuromuscular control of skeletal muscles based on in vivo, high-density MMG data. For this purpose, we will design the first high-density MMG prototypes with up to 96 OPMs and develop custom calibration techniques. We will record magnetic fields induced by contracting skeletal muscles at the highest resolution ever measured. Such data, combined with the advanced computational musculoskeletal system models, will allow us to derive robust and reliable source localisation and separation algorithms. This will provide us with unique input for subject-specific neuromuscular models. We will demonstrate the superiority of the data over existing techniques with two applications; signs of ageing and neuromuscular disorders and show that it is possible to transfer these methodologies to clinical applications.
Hopefully many to come in the next 5 years.
Vera Tahedl
Administrative Associate (Röhrle group)
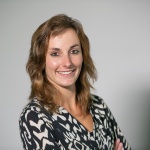
Sina Schorndorfer
Coordinator GRK 2198 / SPP2311