The human spine is a column of alternating soft-tissue and hard-tissue segments. The hard-tissue segments are bones referred to a vertebrae. Accidents may cause painful fractures of these vertebrae. Vertebroplasty is a medical procedure in which bio-compatible cement is injected into damaged vertebral bodies. This immediately causes pain to cease. Further, the injected bone cement undergoes curing, which immobilizes bone fragments and fractures, stabilizing the vertebral body. This outcome requires an even distribution of bone cement within the vertebral body.
Currently, vertebroplasty procedures are performed by hand, guided only by X-ray monitoring and intuition. A common and undesired complication is the leakage of bone cement into extra-vertebral tissue. Then, mechanical stabilization of the damaged bone cannot occur. Such an outcome is difficult to predict, since the involved materials – including the bone cement – exhibit unintuitive material behavior. However, predicting and preventing such complications is paramount to patient care. In particular, the injection of bone cement is always irreversible and – in case of undesired outcomes – incorrigible.
Numerical models and simulations will be meaningful tools regarding the assessment of operating parameters towards the prevention of complications during vertebroplasty. In this project, mathematical and numerical models have already been derived and implemented, describing and simulating the process of bone cement injection into porous bone structures. Theses models are macro-scale models based on the well-known Theory of Porous Media.
The main challenges of the project are:
- Development of subject-specific vertebroplasty models
- Integration of pore-scale processes into macro-scale models
- Experimental validation (in-vitro) of numerical models and simulations (in-silico)
This project is funded by the SFB1313. Experiments are performed in collaboration with the AO Research Institute Davos.
Partial and/or complete tooth loss strongly influences quality of life and its prevalence is likely to increase given an aging word-wide population. Undesired mechanical loading is a major factor in dental implant failure, which may arise due to improper implant selection or design and/or improper osseointegration. As such, a better understanding of the local deformation and loading of dental implants and surrounding dental structures may lead to better and more robust dental implant designs.
Our research aims to build a virtual masticator which realistically predicts the local deformations within the dental structures during natural chewing, biting and clenching motions. The use of physiologically realistic boundary conditions leads to more realistic predictions of the local deformations and loading of the teeth/implants and surrounding dental structures and ultimately leads to a better understanding of dental implant design and dental pathologies.
Research focus areas are:
- Developping novel methods to determine patient specific, in-vivo, biting forces and occlusal load maps
- Assist dental implant design under realistic occlusal loads
- In collaboration with Fraunhofer IPA (Biomechatronic Systems), the use of a chewing robot with a food repositioning system capable of simulating mastication for validation and food-stuff development
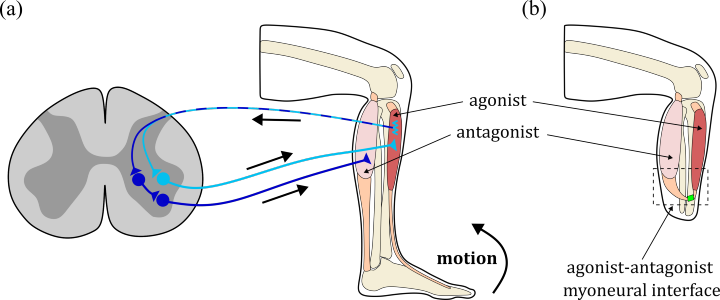
The musculoskeletal and the nervous system exchange information to enable human movement. For example, aognist and antagonist muscles are mechanically coupled by acting on the same joint and additionally exchange sensory information. Conventional amputations destroy the mechanical link between residual muscles and therefor also heavily impair the exchange of sensory information. An emerging surgical approach, the agonist-antagonist myoneural interface (AMI) aims to overcome these drawbacks by mechanically connecting the residual muscles [Herr & Carty 2021]. The AMI is a complex procedure and requires a careful pre-surgery evaluation and planning, for example, to decide which muscles are suitable and how exactly their mechanical connection should be designed in surgery. We pursue a co-design approach for the development of a simulation-based optimization framework (in silico laboratory) to support the identification of optimal physiological and anatomical parameters for the surgical amputation procedure [Homs-Pons et al. 2024]. We specifically aim at advances in:
-
modelling the feedback mechanisms in realistic skeletal muscle pairs
-
their efficient simulation utilising HPC resources (in cooperation with IPVS)
-
the development of Bayesian techniques for parameter inference and optimisation using efficient and accurate surrogate models (in cooperation with IANS).
This project is part of SPP2311 and we closely collaborate with University of Twente and Hannover Medical School.