Impressions
Research Tracks
There are no reliable methods today which allow in vivo and in motu measuring of the loads in the internal structures of the human body. It happens because implanting any sensor device is in the vast majority of conceivable cases both ethically unacceptable and way too impeding not to disturb natural movement. Consequently, predictive biomechanical modelling is required to estimate internal loads.
We use a broad set of classical biomechanical methods and approaches in this research track. The main focus is the development of validated musculoskeletal human body models, with the overall aim to determine consistent scenarios of the mechanical loads that act on all load-constitutive structures of the human body. To solve this task, we develop an in-house code demoa, which solves ODEs and has animation capabilities for muscle-driven systems.
Another speciality in this track is a coupling of our muscle modelling and motion generation methods with commercially available finite element full human body models (THUMS, GHBMC, VIVA OpenHBM) to use their validated assessment capability for automotive and ergonomics research. The unifying approach in this track is our modelling tool calcman, which is under constant development. Its features are a comprehensive dataset of body parts including material properties, full geometrical scalability, and output parser for several simulation packages.
Cooperations and funding: Our work is funded by EU Horizon 2020 programme, different Ministries of Baden-Wuerttemberg State and is supported by industry. In our projects we collaborate with such industry partners as Audi AG, Daimler AG, Mercedes-Benz Cars, Robert Bosch GmbH, Dr. Ing. h.c. F. Porsche AG, Volkswagen AG, ZF Friedrichshafen, Autoliv Deutschland and many others. Among our University partners we can name Ludwig Maximilian University of Munich, RWTH Aachen University, Graz University of Technology, University of Strasbourg. In the OSCCAR (Future Occupant Safety for Crashes in Cars) Project (http://osccarproject.eu/) we work with more than 18 partners from over the world.
Martynenko, O.; Neininger, F. T. & Schmitt, S.: Development of a Hybrid Muscle Controller for an Active Finite Element Human Body Model in LS-DYNA Capable of Occupant Kinematics Prediction in Frontal and Lateral Maneuvers. In: Proceedings of the 26th International Technical Conference on the Enhanced Safety of Vehicles (ESV)., 2019, S. 1--12
N. Brown, D. Bubeck, D. F. B. Häufle, J. Weickenmeier, E. Kuhl, W. Alt, and S. Schmitt. “Weekly Time Course of Neuro-Muscular Adaptation to Intensive Strength Training”. In: Frontiers in Physiology 8 (2017), p. 329. ISSN: 1664-042X. DOI: 10.3389/fphys.2017.00329
C. Kleinbach, O. Martynenko, J. Promies, D. F. B. Häufle, J. Fehr, and S. Schmitt. “Implementation and validation of the extended Hill-type muscle model with robust routing capabilities in LS-DYNA for active human body models”. In: BioMedical Engineering OnLine 16.1 (Sept. 2017), p. 109. ISSN: 1475-925X. DOI: 10.1186/s12938-017-0399-7
O. Roehrle, M. Sprenger, and S. Schmitt. “A two-muscle, continuum-mechanical forward simulation of the upper limb”. In: Biomechanics and Modeling in Mechanobiology 16(3) (2017), pp. 743–762. DOI: 10.1007/s10237-016-0850-x
The evolution of living beings is driven by stochastically induced genetic mutations interplaying with the physical properties and constraints that form and encompass the organism. Yet, questions remain, at least regarding which properties and constraints are the essential ones -- that is, those which can causally explain the phenomenon of life in focus. In this evolutionary context of biological locomotion, we have contributed reductionist biomechanical models of legged terrestrial animal locomotion. Over the years, we have worked on several issues: (i) three-segment leg design, (ii) predicting the mechanical load sharing among legs that contact a substrate at the same time, (iii) identifying possible control parameters to maximise substrate slopes relative to gravity, which can be negotiated by multi-legged animals, (iv) examining the contribution of hydraulics to leg actuation (in spiders), (v) the interrelation of environmental living and physiological conditions, biomechanical body-leg design, and environment-specific solutions to legged locomotion in arthropods, and (vi) identifying geometrical-anatomical and muscle-mechanical parameters of a leg that critically determine maximum running speed across all animal species and the whole range of body sizes (legged speed allometry). Please see also Computer Synthesis of Human Motion.
Cooperations and funding: This research field has been inspired by and emerged from co-working with Reinhard Blickhan (Friedrich-Schiller-Universität, Jena) at whom's research group some of us have received major cuts and
polishes in our scientific approaches. The cooperation partners are Tom Weihmann, Daniel Häufle and Robert Rockenfeller. Funding has been provided by DFG (MU1766/1-3, SE1042/4-1, SI841/2-3, BL-236/14-1, WE4664/2-1, WE4664/5-1, EXC310/1,2, SCHM2392/5-1,2), Deutsche Gesetzliche Unfallversicherung (DGUV) and Volkswagenstiftung (1/78 578).
M. Hammer, M. Günther, D. F. Häufle, and S. Schmitt. “Tailoring anatomical muscle paths: a sheath-like solution for muscle routing in musculo-skeletal computer models”. In: Mathematical Biosciences 311 (2019), pp. 68–81. DOI: 10.1016/j.mbs.2019.02.004
T. Weihmann, H. H. Goetzke, and M. Günther. “Requirements and limits of anatomy-based predictions of locomotion in terrestrial arthropods with emphasis on arachnids”. In: Journal of Paleontology 89.6 (2015), pp. 980–990. DOI: 10.1017/jpa.2016.33
S. W. Lipfert, M. Günther, D. Renjewski, and A. Seyfarth. “Impulsive ankle push-off powers leg swing in human walking”. In: The Journal of Experimental Biology 217.Pt 8 (2014). [with Correction], pp. 1218–1228. DOI: 10.1242/jeb.097345
M. Günther and T. Weihmann. “Climbing in hexapods: A plain model for heavy slopes”. In: Journal of Theoretical Biology 293 (2012), pp. 82–86. DOI: 10.1016/j.jtbi.2011.10.011
R. Blickhan, A. Seyfarth, H. Geyer, S. Grimmer, H. Wagner, and M. Günther. “Intelligence by mechanics”. In: Philosophical Transactions of the Royal Society A 365.1850 (2007), pp. 199–220. DOI: 10.1098/rsta.2006.1911
Motion in biology serves many functions. Most importantly, it impacts the respective species evolutionaly fitness. The variety and adaptivity to specific needs is fascinating throughout the animal kingdom - humans included. At a closer look, biological motion control seems to be hierarchically organised and is driven by a layered structure of different elements and parts, namely, reflex loops, spinal cord, cerebellum, motor cortex, etc. We are trying to understand the contributions of these individual parts by synthesising meaningful and observable motion using biophysical models in computer simulations and mechanical experiments in real life.
Cooperations and funding: We have been funded by Baden-Württemberg Stiftung Neurorobotics, Tübingen-Stuttgart research alliance "System Mensch" (Az: 33-7533.-30-20/7/2), DFG (EXC310, EXC2075), and DFG (GRK2198 "Soft Tissue Robotics"). Our cooperation partners are Daniel Häufle (U Tübingen) and Matthias Müller (U Hannover).
S. Brändle, S. Schmitt, and M. A. Müller. “A systems-theoretic analysis of low-level human motor control: application to a single-joint arm model”. In: Journal of Mathematical Biology 80.4 (2020), pp. 1139–1158. DOI: 10.1007/s00285-019-01455-z
I. Wochner, D. Driess, H. Zimmermann, D. F. B. Häufle, M.Toussaint, and S. Schmitt. “Optimality Principles in Human Point-to-Manifold Reaching Accounting for Muscle Dynamics”. In: Frontiers in Computational Neuroscience 14 (2020), p. 38. DOI: 10.3389/fncom.2020.00038
D. F. B. Häufle, B. Schmortte, H. Geyer, R. Müller, and S. Schmitt. “The Benefit of Combining Neuronal Feedback and Feed-Forward Control for Robustness in Step Down Perturbations of Simulated Human Walking Depends on the Muscle Function”. In: Frontiers in Computational Neuroscience 12 (2018), p. 80. ISSN: 1662-5188. DOI: 10.3389/fncom.2018.00080
D. Suissa, M. Günther, A. Shapiro, I. Melzer, and S. Schmitt. “On Laterally Perturbed Human Stance: Experiment, Model, and Control.” In: Applied Bionics and Biomechanics (2018), p. 4767624. ISSN: 1176-2322. DOI: 10.1155/2018/4767624
A. Bayer, S. Schmitt, M. Günther, and D. Häufle. “The influence of biophysical muscle properties on simulating fast human arm movements”. In: Computer Methods in Biomechanics and Biomedical Engineering 20.8 (2017), pp. 803–821. DOI: 10.1080/10255842.2017.1293663
K. Ghazi-Zahedi, D. F. Häufle, G. Montúfar, S. Schmitt, and N. Ay. “Evaluating morphological computation in muscle and dc-motor driven models of hopping movements”. In: Frontiers in Robotics and AI 3 (2016), p. 42. DOI: 10.3389/frobt.2016.00042
M. Günther and H. Wagner. “Dynamics of quiet human stance: computer simulations of a triple inverted pendulum model”. In: Computer Methods in Biomechanics and Biomedical Engineering 19.8 (2016), pp. 819–834. DOI: 10.1080/10255842.2015.1067306
D. F. B. Häufle, M. Günther, G. Wunner, and S. Schmitt. “Quantifying control effort of biological and technical movements: An information-entropy-based approach”. In: Physical Review E 89 (1 2014), p. 012716. DOI: 10.1103/PhysRevE.89.012716
Since the pioneering work by H. Hatze (1976), who introduced the use of Hill-type muscle models in biomechanical, neuro-musculo-skeletal rigid-body computer models, the elasticity of aponeurosis and tendon material is taken into account in terms of an elastic force element attached in series to the actively contracting force-velocity element representing fibre material properties according to Hill's relation. The force equilibrium between the active force-velocity relation---also depending on the force-length relation and the chemical state, i.e., activity of the fibre material---and the tendon force is the mechanical core of any Hill-type muscle model, and determines the muscle's contraction velocity. This mechanically determined connection is termed the `contraction dynamics' of the fibre material. The contraction dynamics of muscle fibres is strongly coupled to the electro-chemical dynamics of changing fibre activity, that is, the activation dynamics. We are focused on incorporating fibre-internal physiological processes like fatigue, caused by various mechanisms, and myosin-titin-actin interactions inducing history effects in force generation. As Hill-type muscle models constitute a core part of our biophysical computer models of body movements, and, moreover, also serve as blueprints for real-world artificial muscles in biorobotics, we have worked on enhancing Hill-type muscle models. For example, we have added a model of mechanical damping within the aponeurosis/tendon material, which had been assumed to be ideally elastic in Hill-type models since then. Also, some state-dependencies of the parameters making up the Hill relation, which had originally been assumed to be constants, have been reformulated. Furthermore, we have investigated both the electro-mechanical delay---that is, force changes in response to changes in neural stimulation input to the model---and the characteristics of the fibres' force-length relation and their impact on movement simulations, and our `workhorse' Hill-type muscle model has been polished to function well for any contractile state, including stretching of the fibres (i.e., working eccentrically).
Additionally, we have developed a very reduced muscle model that consists of just four force elements representing the basic mechanical structure of a single cross-bridge. The model can explain at once the mechanical power and heat output during concentric steady-state contractions of skeletal muscle as well as early responses to rapid steps in muscle length or force. An essential part of the findings facilitated by this model approach is, firstly, that just two model parameters are free to be chosen for fitting the characteristics measured in the non-steady-state responses to rapid steps, whereas the other six parameters reflect knowledge from literature, including microscopic cross-bridge geometry and force characteristics that point to Coulomb repulsion between different cross-bridge regions being the elementary drive. Secondly, the macroscopic, steady-state characteristics of contracting muscle fibres (Hill's force-velocity relation) can be derived from exactly the same model, with (i) the force simply scaled up from cross-bridge level, (ii) assuming that ATP hydrolysis adds, in steady-states, to (one of the two) damping (coefficients) as a second dissipative process beyond passive friction, and (iii) using the contribution of the fibre-internal elasticity to external contraction velocity as one of just two free parameters to fit the steady-state characteristics.
Cooperations and funding: Our partners are Tobias Siebert (U Stuttgart) and Robert Rockenfeller (Universität Koblenz-Landau). This research track has been supported by DFG (MU1766/1-1,2,3, BL236/11-1, SI841/2-3, EXC310/1,2, SCHM2392/5-1,2, EXC2075), Land Baden-Württemberg (Az: 33-7533.-30-20/7/2), and Berufsgenossenschaft Nahrungsmittel und Gastgewerbe (BGN, Mannheim, Germany).
M. Günther, D. F. Häufle, and S. Schmitt. “Corrigendum to “The basic mechanical structure of the skeletal muscle machinery: One model for linking microscopic and macroscopic scales” [Journal ofTheoretical Biology 456 (2018) 137–167]”. In: Journal ofTheoretical Biology 488 (2020), p. 110143. DOI: 10.1016/j.jtbi.2019.110143
D. F. B. Häufle, J. Siegel, S. Hochstein, A. Gussew, S. Schmitt, T. Siebert, R. Rzanny, J. R. Reichenbach, and N. Stutzig. “Energy expenditure of dynamic submaximal human plantarflexion movements: model prediction and validation by in-vivo magnetic resonance spectroscopy”. In: Frontiers in Bioengineering and Biotechnology 8.622 (2020).
R. Rockenfeller, M. Günther, N. Stutzig, D. F. B. Häufle, T. Siebert, S. Schmitt, K. Leichsenring, M. Böl, and T. Götz. “Exhaustion of skeletal muscle fibers within seconds: incorporating phosphate kinetics into a Hill-type model”. In: Frontiers in Physiology 11 (2020), p. 306. DOI: 10.3389/fphys.2020.00306 .
S. Schmitt, M. Günther, and D. F. B. Häufle. “The dynamics of the skeletal muscle: A systems biophysics perspective on muscle modeling with the focus on Hill-type muscle models”. In: GAMM-Mitteilungen 0 (2019), e201900013. ISSN: 0936-7195. DOI: 10.1002/gamm.201900013 .
M. Günther, D. F. Häufle, and S. Schmitt. “The basic mechanical structure of the skeletal muscle machinery: One model for linking microscopic and macroscopic scales”. In: Journal of Theoretical Biology 456 (2018), pp. 137–167. ISSN: 0022-5193. DOI: 10. 1016 / j.jtbi.2018.07.023 .
R. Rockenfeller and M. Günther. “Inter-filament spacing mediates calcium binding to troponin: A simple geometric-mechanistic model explains the shift of force-length maxima with muscle activation”. In: Journal of Theoretical Biology 454 (2018), pp. 240–252. DOI: 10.1016/j.jtbi.2018.06.009 .
R. Rockenfeller and M. Günther. “Hill equation and Hatze’s muscle activation dynamics complement each other: enhanced pharmacological and physiological interpretability of modelled activity-pCa curves”. In: Journal of Theoretical Biology 431 (2017), pp. 11–24. DOI:10.1016/j.jtbi.2017.07.023 .
R. Rockenfeller and M. Günther. “How to model a muscle’s active force-length relation: A comparative study”. In: Computer Methods in Applied Mechanics and Engineering 313 (2017), pp. 321–336. DOI: 10.1016/j.cma.2016.10.003 .
R. Rockenfeller, M. Günther, S. Schmitt, and T. Götz. “Corrigendum to Comparative Sensitivity Analysis of Muscle Activation Dynamics”. In: Computational and Mathematical Methods in Medicine 2017 (2017), p. 2.
R. Rockenfeller and M. Günther. “Extracting low-velocity concentric and eccentric dynamic muscle properties from isometric contraction experiments”. In: Mathematical Biosciences 278 (2016). [with Corrigendum], pp. 77–93. DOI: 10.1016/j.mbs.2016.06.005 .
R. Rockenfeller, M. Günther, S. Schmitt, and T. Götz. “Comparative sensitivity analysis of muscle activation dynamics”. In: Computational and Mathematical Methods in Medicine (2015). [with Corrigendum], 585409 (16pp). DOI: 10.1155/2015/585409 .
D. F. B. Häufle, M. Günther, A. Bayer, and S. Schmitt. “Hill-type muscle model with serial damping and eccentric force-velocity relation”. In: Journal of Biomechanics 47.6 (2014), pp. 1531–1536. DOI: 10.1016/j.jbiomech.2014.02.009 .
E. V. Rosenfeld and M. Günther. “An enhanced model of cross-bridge operation with internal elasticity”. In: European Biophysics Journal 43.4-5 (2014), pp. 131–141. DOI: 10.1007/s00249-014-0947-z .
M. Günther and S. Schmitt. “A macroscopic ansatz to deduce the Hill relation”. In: Journal of Theoretical Biology 263.4 (2010), pp. 407–418. DOI: 10.1016/j.jtbi.2009.12.027 .
M. Günther, S. Schmitt, and V. Wank. “High-frequency oscillations as a consequence of neglected serial damping in Hill-type muscle models”. In: Biological Cybernetics 97.1 (2007), pp. 63–79. DOI: 10.1007/s00422-007-0160-6 .
This research track is one of our oldest, dating back to early days at University Tübingen, Prof. Hanns Ruder. Realising purely synthetic, dynamically stable neuro-musculo-skeletal computer simulations of human walking in two dimensions (2d; in the sagittal plane) can be seen a first, measurable accomplishment. Although an intermediate step had been made in achieving three-dimensional (3d) walking simulations using a robotic (torque-driven) approach, the huge step to having eventually at hand a solely muscle-driven neuro-musculo-skeletal 3d-model of the human for the pure computer synthesis of walking movements has, however, not been made so far. In the meantime, though, we have accumulated a lot of biomechanical knowledge as well as modelling experience and absolutely essential computer model tools and structures: We solved the muscle routing/redirection problem in 3d, we are enhancing coupled models of muscle contraction and activation, and we have looked experimentally into crucial characteristics of human walking dynamics, all of this often in cooperations with long-lasting histories. It is, thus, very likely that we are going to accomplish the major step to a 3d computer model in the upcoming years.
Cooperations and funding: We have been funded by the DFG (EXC310/1,2, EXC2075). Over the years, our partners have been Susanne Lipfert und Daniel Renjewski (both TU München) and Robert Rockenfeller (Universität Koblenz-Landau). Arnim Henze developed the forerunner 'dysim' of our in-house simulation code 'demoa' and was promoted, supervised by Hanns Ruder (Theoretische Astrophysik, Tübingen, Germany), by virtue of his doctoral thesis in which he demonstrated how to simulate 3d-walking by torque drives, based on the robotic control approach 'virtual model control (VMC)'.
M. Hammer, M. Günther, D. F. Häufle, and S. Schmitt. “Tailoring anatomical muscle paths: a sheath-like solution for muscle routing in musculo-skeletal computer models”. In: Mathematical Biosciences 311 (2019), pp. 68–81. DOI: 10.1016/j.mbs.2019.02.004
A. Bayer, S. Schmitt, M. Günther, and D. Häufle. “The influence of biophysical muscle properties on simulating fast human arm movements”. In: Computer Methods in Biomechanics and Biomedical Engineering 20.8 (2017), pp. 803–821. DOI: 10.1080/10255842.2017.1293663
T. K. Rupp, W. Ehlers, N. Karajan, M. Günther, and S. Schmitt. “A forward dynamics simulation of human lumbar spine flexion predicting the load sharing of intervertebral discs, ligaments, and muscles”. In: Biomechanics and Modeling in Mechanobiology 14.5 (2015), pp. 1081–1105. DOI: 10.1007/s10237-015-0656-2
Inherent to ontogeny is adaptation and learning. Biological systems exploit their own body characteristics and environmental interactions of all kind. Still today, the interdependence between physical state dependency and central nervous pathways are nourishment for modern research. We use a system dynamics perspective on the biological organism and focus on the learning process to generate meaningful motion. We showed that it is possible to apply and develop artificial learning techniques to accomplish specific motion tasks. We ask for generalisation of learning in the interaction with the environment and the body structure. Thereby, assumed optimality of biological motion helps to assess technical solutions.
Cooperations and funding: We have been funded by Baden-Württemberg Stiftung Neurorobotics, Tübingen-Stuttgart research alliance "System Mensch" (Az: 33-7533.-30-20/7/2), and DFG (EXC310, EXC2075). Our cooperation partners are Marc Toussaint (TU Berlin), Daniel Häufle (U Tübingen), and Alexander Spröwitz (MPI Stuttgart).
I. Wochner, D. Driess, H. Zimmermann, D. F. B. Häufle, M.Toussaint, and S. Schmitt. “Optimality Principles in Human Point-to-Manifold Reaching Accounting for Muscle Dynamics”. In: Frontiers in Computational Neuroscience 14 (2020), p. 38. DOI: 10.3389/fncom.2020.00038 .
D. Suissa, M. Günther, A. Shapiro, I. Melzer, and S. Schmitt. “On Laterally Perturbed Human Stance: Experiment, Model, and Control.” In: Applied Bionics and Biomechanics (2018), p. 4767624. ISSN: 1176-2322. DOI: 10.1155/2018/4767624 .
D. Driess, S. Schmitt, and M. Toussaint. “Active Inverse Model Learning With Error and Reachable Set Estimates”. In: 2019 IEEE/RSJ International Conference on Intelligent Robots and Systems (IROS). 2019, pp. 1826–1833. DOI: 10.1109/IROS40897.2019.8967858 .
D. Driess, H. Zimmermann, S. Wolfen, D. Suissa, D. Haäufle, D. Hennes, M. Toussaint, and S. Schmitt. “Learning to Control Redundant Musculoskeletal Systems with Neural Networks and SQP: Exploiting Muscle Properties”. In: 2018 IEEE International Conference on Robotics and Automation (ICRA). 2018, pp. 6461–6468. DOI: 10.1109/ICRA.2018.8463160 .
K. Ghazi-Zahedi, D. F. Häufle, G. Montúfar, S. Schmitt, and N. Ay. “Evaluating morphological computation in muscle and dc-motor driven models of hopping movements”. In: Frontiers in Robotics and AI 3 (2016), p. 42. DOI: 10.3389/frobt.2016.00042 .
D. F. B. Häufle, M. Günther, G. Wunner, and S. Schmitt. “Quantifying control effort of biological and technical movements: An information-entropy-based approach”. In: Physical Review E 89 (1 2014), p. 012716. DOI: 10.1103/PhysRevE.89.012716 .
Robots are attractive from many viewpoints. We build robots to validate our computer moduls, to see our theory in real life, and to have our hands on a real system. In computational approaches, sometimes, the solution of a hard problem looks easy, but technically it is not feasible with available state of the art technology. Building physical models untangles additional challenges evolution faced and often solved. In recent years, we have focussed on building physical models of biological muscle and couple these to an artifical skeleton to build leg and arm robots.
Cooperations and funding: We have been funded by Baden-Württemberg Stiftung Neurorobotics, Tübingen-Stuttgart research alliance "System Mensch" (Az: 33-7533.-30-20/7/2), and DFG (EXC310, EXC2075). Our cooperation partners are Marc Toussaint (TU Berlin), Daniel Häufle (U Tübingen), and Alexander Spröwitz (MPI Stuttgart).
D. Driess, H. Zimmermann, S. Wolfen, D. Suissa, D. Häufle, D. Hennes, M. Toussaint, and S. Schmitt. “Learning to Control Redundant Musculoskeletal Systems with Neural Networks and SQP: Exploiting Muscle Properties”. In: 2018 IEEE International Conference on Robotics and Automation (ICRA). 2018, pp. 6461–6468. DOI: 10.1109/ICRA.2018.8463160 .
S. Wolfen, J. Walter, M. Günther, D. F. B. Häufle, and S. Schmitt. “Bioinspired pneumatic muscle spring units mimicking the human motion apparatus: benefits for passive motion range and joint stiffness variation in antagonistic setups”. In: 2018 25th International Conference on Mechatronics and Machine Vision in Practice (M2VIP). 2018, pp. 1–6. DOI: 10.1109/M2VIP.2018.8600913 .
D. F. B. Häufle, M. Günther, R. Blickhan, and S. Schmitt. “Can quick release experiments reveal the muscle structure? A bionic approach”. In: Journal of Bionic Engineering 9.2 (2012), pp. 211–223. DOI: 10.1016/S1672-6529(11)60115-7.
D. F. B. Häufle, M. Günther, R. Blickhan, and S. Schmitt. “Proof of concept: model based bionic muscle with hyperbolic force-velocity relation”. In: Applied Bionics and Biomechanics 9.3 (2012), pp. 267–274. DOI: 10.3233/ABB-2011-0052 .
D. F. B. Häufle, M. D. Taylor, S. Schmitt, and H. Geyer. “A clutched parallel elastic actuator concept: Towards energy efficient powered legs in prosthetics and robotics”. In: 2012 4th IEEE RAS EMBS International Conference on Biomedical Robotics and Biomechatronics (BioRob). 2012, pp. 1614–1619. DOI: 10.1109/BioRob.2012.6290722.
S. Schmitt, D. F. B. Häufle, R. Blickhan, and M. Günther. “Nature as an engineer: one simple concept of a bio-inspired functional artificial muscle”. In: Bioinspiration & Biomimetics 7.3 (2012), 036022 (9pp). DOI: 10.1088/1748-3182/7/3/036022 .
The human (and animal) spine is a sub-organism of the whole body, which represents a crucial mechanical structure of each body's skeleton. Although a major focus in health research for a very long time, still, basic physical interactions (independent of all other unexplained phenomena in, e. g., microbiology) are partly known or unknown. For example, the contribution of spinal ligaments in living creatures to passive and active trunk stabilisation cannot fully be explained, still today. We focus on a reduced approach to spine mechanics, and study non-linear dynamics of signficant load bearing structures of this sub-organism. The physical state dependencies, actuators and sensor dynamics, together with present central nervous feedback and motor commands are used to model a fully dynamic, coupled biological system. Nevertheless, we strive to merge abstract multi-body approaches with geometry-detailed finite element description to leverage advantages of both worlds in one modelling and simulation approach.
Cooperations and funding: We have been funded by the DFG (EXC310/1,2, EXC2075), DAAD, Deutsche Gesetzliche Unfallversicherung and ARC. Cooperation partners are Falk Moerl (APZ Erfurt), Brigitte Benda-Schäfer (Stuttgart), Farzam Vazifehdan (Diakonieklinikum Stuttgart) and Peter Pivonka and Paige J. Little (Biomechanics and Spine Research Group at QUT / Brisbane, Australia).
B. Haasdonk, T. Wenzel, G. Santin, and S. Schmitt. Biomechanical surrogate modelling using stabilized vectorial greedy kernel methods. 2020. arXiv: 2004.12670 [math.NA] .
F. Mörl, M. Günther, J. M. Riede, M. Hammer, and S. Schmitt. “Loads distributed in vivo among vertebrae, muscles, spinal ligaments, and intervertebral discs in a passively flexed lumbar spine”. In: Biomechanics and Modeling in Mechanobiology (published online) (2020). DOI: 10.1007/s10237-020-01322-7 .
T. K. Rupp, W. Ehlers, N. Karajan, M. Günther, and S. Schmitt. “A forward dynamics simulation of human lumbar spine flexion predicting the load sharing of intervertebral discs, ligaments, and muscles”. In: Biomechanics and Modeling in Mechanobiology 14.5 (2015), pp. 1081–1105. DOI: 10.1007/s10237-015-0656-2 .
N. Karajan, O. Röhrle, W. Ehlers, and S. Schmitt. “Linking continuous and discrete intervertebral disc models through homogenisation”. In: Biomechanics and Modeling in Mechanobiology 12.3 (June 2013), pp. 453–466. ISSN: 1617-7940. DOI: 10.1007/s10237-012-0416-5 .
Biological locomotion is driven by skeletal muscles. Muscle masses are suspended compliantly at the skeleton. Any (human or animal) leg that impacts with the ground during running, jumping, or hopping, and any limb that boxes, bangs, or bats something induces shock waves running through the limb and the body. These impact-induced, damped oscillations also superpose the driving muscle contractions themselves. Until today, muscle modelling has been neglecting, on a very broad scale, that the muscle fibres in an anatomically intact, in-vivo, in-situ, and in-motu muscle customarily have to contract against their own mass inertia. Accordingly, state-of-the-art muscle models for describing muscle contraction are, expressed in a mathematical language, of first order: they consist of ordinary first-order differential equations. If mass inertia is taken into account, the equations covering muscle contraction dynamics turn to being of second order, namely, regularly mechanical character. Along this research track, we have firstly introduced inertia in a plain, straight-forward way, namely, letting classical Hill-type model elements (see other research track) interact with point masses evenly distributed between them, and examining, in numerical computer experiments, the contractile response in dynamic contractions of a maximally activated muscle with one of its ends released free. Secondly, in a recent study, we have shown that the muscle's own inertia is likely to be the very biomechanical property that crucially limits the maximum speed to be achieved by bigger animals (like humans) in legged terrestrial locomotion (see also `Human and animal locomotion').
Cooperations and funding: This research track has been funded by the DFG (EXC310/1,2, SI841/2-3, SCHM2392/5-1,2). Cooperation partners are Tobias Siebert (U Stuttgart).
K. B. Christensen, M. Günther, S. Schmitt, and T. Siebert. “Strain in shock-loaded skeletal muscle and the time scale of muscular wobbling mass dynamics”. In: Scientific Reports 7 (2017), 13266 (11pp). DOI: 10.1038/s41598-017-13630-7.
M. Günther, D. F. B. Häufle, O. Röhrle, and S. Schmitt. “Spreading out muscle mass within a Hill-type model: A computer simulation study”. In: Computational and Mathematical Methods in Medicine (2012), 848630 (13pp). DOI: 10.1155/2012/848630 .
S. Schmitt and M. Günther. “Human leg impact: energy dissipation of wobbling masses”. In: Archive of Applied Mechanics 81.7 (2011), pp. 887–897. DOI: 10.1007/s00419-010-0458-z .
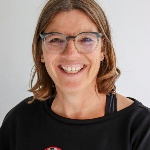
Christine Schreiber
Administrative Associate (Schmitt group)